INTRODUCTION
Muscle size is the morphological basis of determining maximum muscle strength.1 Previous studies suggested that muscl cross-sectional area (CSA) is positively correlated with maximal voluntary strength.2-4 These studies tested relatively simple joint actions such as knee extension and elbow flexion.2-4 Rankin reported abdominal muscle thickness measured by ultrasound and suggested that muscle size may be an indirect measure of force-generating capacity as demonstrated in various muscles.5 However, determining trunk kinematics from a single rigid joint is somewhat different.6 The spine consists of multi-segment joints with multiple attachment sites for the core muscles.7 Also, because there is a wide range of directions for the trunk muscle lines of action, a single core muscle’s CSA tends to simplify a set of numerous muscle slips.8
Panjabi suggested that the active subsystem (muscles and tendons around the spine) plays important roles in stabilizing the spine along with the passive (vertebrae, ligament) and neural (central nervous system and other contributing nerves) subsystems.9 Core muscles comprise a muscular box with the abdominals (rectus abdominis, external oblique, internal oblique, transversus abdominis) in the front, para-spinal muscles (erector spinae, multifidus) in the back, diaphragm as the roof, and pelvic floor musculature as the bottom around the spine.10 Particular attention has been paid to the core muscles because they serve as a muscular corset that works as a unit to stabilize the body and spine.11 Core stability has been defined as the ability to utilize strength and endurance in a functional manner through all planes of motion and action despite changes in the center of gravity.12 Thus, it is believed that core muscles must have sufficient strength and endurance to ensure core stability.10
Measuring core stability is difficult, and no test or measure serves as the gold standard. Many isometric core muscle endurance tests have been used to assess core stability in clinical or field settings.13-15 These tests require minimal inexpensive equipment and are simple to employ in clinical environments. McGill reported the normative values for trunk flexor, extensor, and lateral flexor endurance to quantify core stability in participants from a university community.13 Other studies reported trunk muscle endurance in athletes and firefighters using same protocols.16 The plank endurance test is also used to assess core stability.16,17 Sahrmann suggested the double-leg loading test to assess the ability to control the spine while extending the lower extremities.18 This test originally assessed core stability in five stages.18 Previous studies modified the test to quantify the results using a pressure biofeedback unit.19,20
Training programs for improving core stability often target improving core strength and endurance.10,21,22 Increased muscle size is also an expected effect of training programs.10,23,24. However, the relationship between core muscle CSA and strength, stability, and clinical core test scores has not been identified. Thus, this study aimed to investigate the relationship between core muscle CSA and muscle functions (strength, endurance, stability).
METHODS
Sample size was determined a priori using G-power (version 3.1.3; University of Trier, Trier, Germany) in a pilot study of eight participants. Sample size was calculated with power of 0.80, alpha level of 0.05 (one tailed), and correlation ρ H1 0.35; the results suggested that more than 49 participants were required. Fifty healthy participants (24 men, 26 women) participated in this study. The mean values (SD) of the participants’ age, height, and body mass were 25.1 (2.7) years, 167.7 (8.8) cm, and 65.3 (14.4) kg, respectively. Participants were included if they had no current or past history of low back pain in the last 12 months, previous spinal surgery or fracture, neurological or orthopedic disease, or open abdominal surgery. Participants were excluded if they had contraindications to magnetic resonance imaging (MRI) such as metallic implants, cardiac pacemakers, or claustrophobia. This study was approved by Yonsei University Wonju Institutional Review Board (approval no. 1041849-201904-BM-054-02).
MRI scans were obtained using a 1.5T Magnetom Avanto (Siemens, Erlangen, Germany). T2 axial MRI scans were obtained using repetition time (TR)/echo time (TE)=2100 ms/91 ms, 256 × 156 matrix, field of view (FOV) 400 mm × 325 mm. Ten contiguous 5-mm-thick slices centered on the L3–4 discs were acquired in the relaxed state. Participants were required to hold their breath during the MR scan to avoid movement artifacts. The MRI results were archived for later analyses using a measurement software package on a laptop computer. The CSA of the abdominal muscles (rectus abdominis, lateral abdominal wall) and paraspinal muscles (erector spinae, multifidus) were measured (Figure 1). All of the data analyses were conducted by one assessor to ensure consistency.
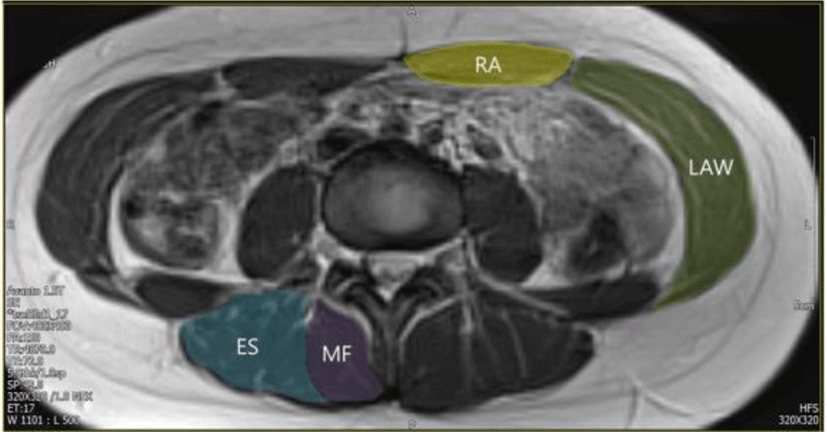
Maximal isometric trunk flexor strength and side bridge strength were measured by a Smart KEMA strength sensor (KOREATECH, Inc., Seoul, Korea).25 Data measured using the sensor were transferred to a tablet PC (Galaxy Tab A6 10.1; Samsung, Inc., Seoul, Korea) via Bluetooth and analyzed using the Smart KEMA application (KOREATECH, Inc.). To measure maximal isometric trunk flexor strength, participants lay in a hook lying position on a table with their feet fixed to the table with an adjustable belt to stabilize the lower limbs during trunk flexion. A belt was connected to the sensor placed on the middle of the sternum. The participants were instructed to sit up and pull the belt with maximum effort. While the participants performed maximal trunk flexion, the sensor measured the maximal isometric strength as both sides of the sensor were pulled by the belts (Figure 2). To measure maximal isometric side bridge strength, the participants lay on their sides with their legs extended. A belt connected to the strength sensor was placed between the top of the iliac crest and the greater trochanter. Participants were instructed to lift their hips off the table with maximum effort while resting on one elbow and their feet. While the participants performed the maximal side bridge task, the sensor measured the maximal isometric strength (Figure 2). The initial tension value for measuring maximal isometric strength was set to 2 kgf in the start position to control the tension. Both sides were measured and the plus value of both sides was used in the data analysis. The participants were asked to maintain maximal isometric strength for 5 s, and the average value was calculated from the middle 3 s. The mean values of three trials were used in the data analyses. A 30-s rest was provided after each trial and a 5-min rest was provided between conditions to prevent muscle fatigue.
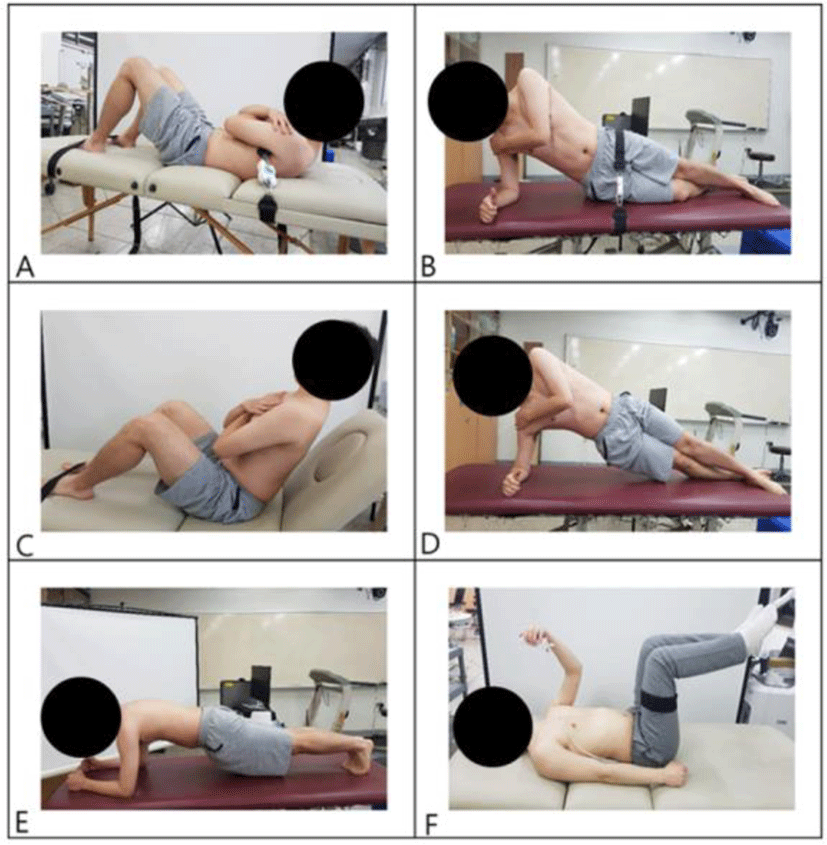
To test trunk flexor endurance, the participants assumed a sit-up posture with the back resting against back supports angled at 55° from the table (Figure 2).13,16 The knees and hips were flexed to 90°. The arms were folded across the chest with the hands placed on the opposite shoulders and the feet were placed under foot straps. The participants were instructed to maintain the body position while the back supports were pulled back 10 cm to begin the test according to previously reported methods.13,16 Failure was determined to occur when any part of the person’s back touched the back supports, the participant requested to stop, or the participant was unable to maintain the correct posture after one verbal warning. To test lateral muscle endurance, the side bridge endurance test was performed (Figure 2). 13,16 The participants lay on their sides with the legs extended. The top foot was placed in front of the lower foot for support. The participants were instructed to support themselves on one elbow and their feet while lifting their hips off the floor to maintain a straight line over their body’s length. The uninvolved arm was held across the chest with the hand placed on the opposite shoulder. The test ended when the participant lost the straight-back posture and the hip returned to the ground, the participant requested to stop, or the participant was unable to maintain the posture after one verbal warning. Both sides were measured and the plus value of both sides was used in the data analysis. For the plank endurance test (Figure 2),16,17 the participants lay prone with the elbows in contact with the ground so the humerus formed a perpendicular line with the horizontal plane directly beneath the shoulders. The elbows were spaced shoulder-width apart in a neutral position and the feet were set with a narrow base without touching. The participants were instructed to raise the pelvis from the floor so that only the forearms and toes were in contact with the floor while maintaining the shoulders, hips, and ankles in a straight line. The test ended when the straight line was no longer maintained, the hips dropped toward the floor, the participant requested to stop, or the participant was unable to maintain the posture after one verbal warning. During the endurance tests, the participants were reminded to maintain the position for as long as possible. To avoid muscle fatigue, a 5-min rest was provided between conditions.
To test the participant’s ability to control the spine, the modified double-leg loading test was performed with maintenance of the abdominal pressure during lower limb movements (Figure 2).18-20 The participants remained in the supine position with their hips and knees flexed to 90°. A Smart KEMA pressure sensor (KOREATECH, Inc.) was set to 40 mmHg and placed below the lordotic curvature of the spine between S1 and L1 with the hips and knees at 90° flexion. A strap with a Smart KEMA motion sensor (KOREATECH, Inc.) was placed on the lateral thigh between the greater trochanter and the knee joint. The participants were instructed to maintain a pressure of 40 mmHg while extending both legs. The angle of both legs lowered (hip extension) measured with a motion sensor was defined as lumbopelvic stability and measured when pressure changed more than 10 mmHg during the lowering of both legs. The mean values of two trials were used for the data analyses for both legs. A 5-min rest was provided between tests to prevent muscle fatigue.
SPSS ver. 25.0 (SPSS, Inc., Chicago, IL, USA) was used for the statistical analysis. The intraclass correlation coefficient (ICC) (3, 1) model was used to test intra-rater measurement reliability. The Kolmogorov–Smirnov test was used to verify the assumption of a normal distribution. The relationship between core muscle CSA and muscle function was examined by Pearson’s correlation coefficient for normally distributed data and by the Spearman rho correlation coefficient for non-normally distributed data. The level of statistical significance was set at α=0.05.
RESULTS
Table 1 shows the core muscle CSA and functions. Maximal isometric trunk flexor strength and side bridge strength were positively correlated with core muscle CSA (r=0.655–0.794; p<0.05) (Table 2) (Figure 3 and 4). The isometric endurance test scores to assess core stability also showed a positive correlation with core muscle CSA (r=0.275–0.594; p<0.05) except for the trunk flexor endurance test, which showed no significant correlation with core muscle CSA. The ICC (3,1) of the maximal isometric trunk flexor strength was 0.970 (95% confidence interval [CI], 0.948–0.983), that of maximal isometric side bridge strength was 0.978 (95% CI, 0.961–0.987), and that of the double-leg loading test was 0.973 (95% CI, 0.954–0.985).
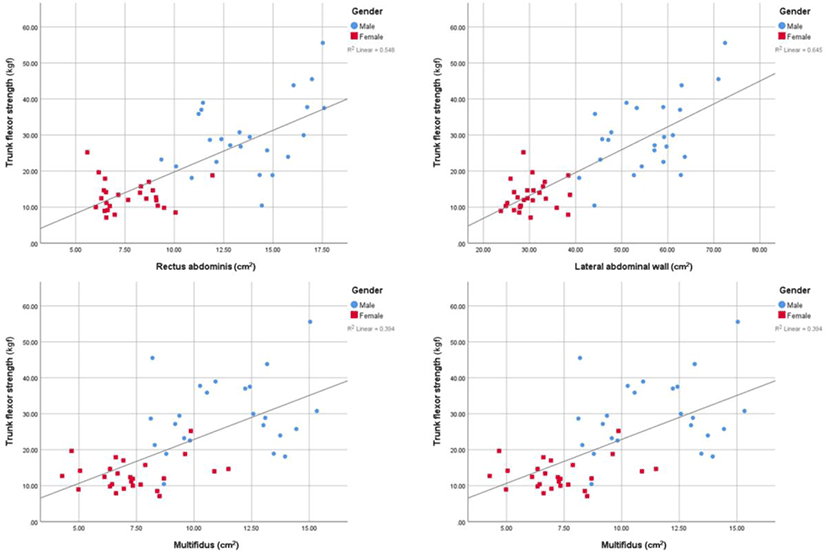
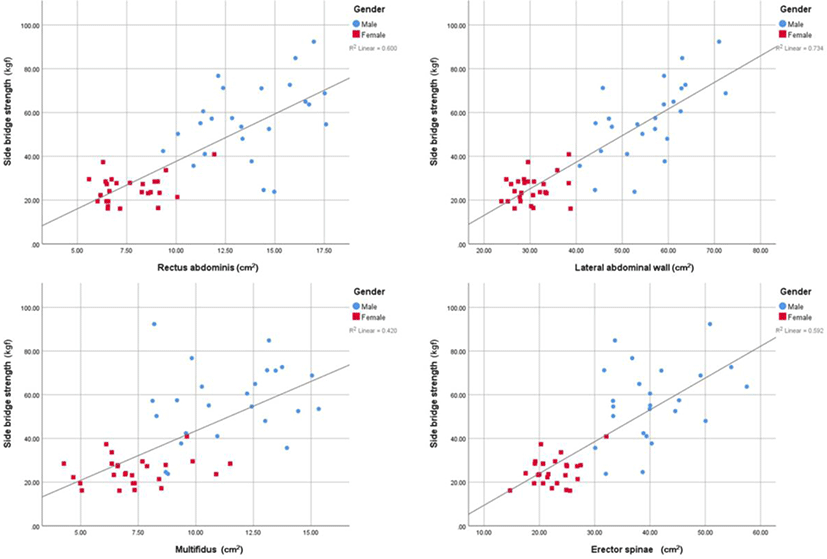
DISCUSSION
Core stability remains a key component in clinical rehabilitation,26 training of competitive athletes,22,27 and training programs of individuals for improving health and physical fitness.28 Trainings to improve core muscle strength and endurance have been used to improve core stability.22,29 This study aimed to investigate the relationship between core muscle CSA and function. In this study, maximal isometric trunk strength and side bridge strength showed moderate to excellent correlations with core muscle CSA (Figures 3 and 4). In contrary, endurance times of the side bridge endurance test and plank endurance test showed relatively lower correlations (fair to good) with core muscle CSA. Furthermore, trunk flexor endurance time had no correlation with core muscle CSA. These findings were consistent with the physiological underpinnings of muscle strength and endurance.
Previous studies reported that muscle CSA is closely related to the muscle’s capacity to generate maximal isometric strength.30,31 Because a larger CSA may indicate the presence of more contractile tissues that can generate more force,32 it would be reasonable if core muscle CSA was significantly correlated with maximal isometric trunk flexor strength and side bridge strength. In this study, the CSA of the agonists (abdominal muscles) and the antagonists (erector spinae and multifidus) were correlated with maximal isometric trunk flexor strength. This is probably because antagonists play an important role in stabilizing the spinal joint during maximal isometric effort.33 Although a single CSA tends to simplify various lines of pull of the core muscles,8 the CSA of the lateral abdominal wall, the plus value of the CSA of the external oblique, internal oblique, and transversus abdominis muscles, showed a higher relationship (r=0.782–0.794) with maximal isometric core strength than one muscle (r=0.648–0.774). Thus, it would be better to perform exercises for not one special core muscle but for balanced core muscles to improve core strength.
In determining muscle endurance, muscle fiber composition may play a more significant role than muscle CSA. Muscle fiber types were classified into type I, type IIA, and type IIx.34 Type I muscles have high amounts of myoglobin and high capillary content, which contribute to greater oxidative capacity.32 Käser et al. reported a significant relationship between trunk extensor endurance test scores and the relative area of the muscle occupied by type I fibers.35 Similarly, Mannion et al. reported that the relative area of the muscle occupied by type I fibers showed a significant correlation with trunk extensor endurance test scores.36 Thus, a larger percentage of type I muscle fibers could contribute to better muscle endurance performance.32,34 However, with same percentage of type I fibers, a muscle with a larger CSA could have greater absolute oxidative capacity than a muscle with a smaller CSA.37 Thus, a larger CSA could increase muscle endurance performance. This might be the reason the plank endurance time and side bridge endurance time showed a significant but lower correlation with core muscle CSA (r=0.275–0.594) than maximal isometric strength.
In this study, unlike the plank endurance time and side bridge endurance time, trunk flexor endurance time showed no correlation with core muscle CSA. There are some possible reasons for this. First, the upper and lower extremities were also used to support the body weight during the side bridge endurance test and plank endurance test.16,38 Thus, both core muscle function and upper and lower extremity muscle function could affect endurance time results.38 Second, the participants had variable anthropometric data, particularly upper body length. The upper body length determined the external load to be maintained in the start position during the trunk flexor endurance test. Chen et al. investigated the effects of different reclined positions in the same participants to create different external loads during the tests.39 Our study results showed that a different external load could vary the flexor endurance time. Third, participants may attempt to prolong the test by subtly flexing their thoracic or cervical spine to compensate for trunk flexor fatigue, presumably to shorten the external moment arm of the head and torso to reduce the trunk flexor torque required to maintain a 60° reclined position.38 Because a muscle with a larger CSA may contain more contractile and connective tissues,40 the increase in these proteins such as collagen, actin, myosin and titin suggests a concurrent increase in muscle stiffness.18,41 Given that existing models focus on active muscle stiffness as the primary control mechanism for spinal stability,42,43 a larger core muscle CSA could contribute to increased mechanical stability of the spine.42 However, in this study, a larger CSA had no practical effect on core muscle endurance. Mcgill suggested that muscular endurance is more important to stability than muscle strength in tasks of daily living.44 On the other hand, performance enhancements such as speed, agility, and power might be expected to occur by increased force generation and muscle stiffness secondary to muscle hypertrophy.10,45 Previous studies reported that athletes have larger core muscle CSA and greater core strength than healthy controls.46,47 Thus, core strength and endurance differ depending on the situations, and both are important contributors to core stability.
There are several limitations to the current study. First, we only measured anatomical CSA. Many studies reported fatty infiltrations in the lumbar extensors of patients with low back pain. A previous study suggested the use of functional CSA to examine contractile tissues.48 However, because the participants in this study were young and had no low back pain, measuring anatomical CSA was appropriate in our investigation of the correlation between core muscle CSA and core muscle test results. Second, because we set a power of 0.80, alpha level of 0.05, and correlation ρ H1 0.35 to calculate the appropriate sample size a priori, the data showing correlation coefficients lower than 0.35 were underpowered to investigate the relationship between core muscle CSA and function. Thus, the relationship between CSA of the multifidus muscle and core muscles test scores was underpowered. Also, the relationship between the CSA of the lateral abdominal wall and the double-leg loading test scores was underpowered. Third, we measured core muscle function isometrically. Because muscle function could be affected by muscle contraction factors such as isometric, isokinetic, or dynamic contraction (concentric and eccentric), further investigations of the relationship between muscle size and isokinetic or dynamic muscle function are needed.
CONCLUSIONS
In conclusion, we noted significant correlations between core muscle CSA and function. However, only core strength showed moderate to excellent correlation with core muscle CSA. These findings indicate that core training to achieve muscle hypertrophy could lead to improved core strength but not core endurance.