INTRODUCTION
Core stability is considered an essential element of musculoskeletal recovery from injury and enhanced sports performance.1–5 A lack of core stability increases the risk of injury. Low back pain patients have weak core muscles or abnormal activation thereof.6–8 Sports performance is also related to core stability. During cycling, when the core muscles become fatigued, the optimal lower extremity alignment for power production is not maintained.9 In addition, after muscular workouts, cool-down programs with core stability exercises tend to enhance postural control. Therefore, incorporating core stability into rehabilitation and training programs is critical for pain and injury prevention, as well as to improve performance.
To determine the appropriate intensity of the rehabilitation or training program, proper evaluation is required. The Sahrmann Core Stability Test (SCST) has been widely used to objectively evaluate core stability.10–12 In the SCST, the subject is required to move the lower extremities while controlling the lumbopelvic segment. To achieve consistent test results, the movement should accurately follow the SCST instructions; additionally, any compensatory movement should be controlled for. Therefore, the examiner must be well-educated and experienced in SCST administration.
Recently, our group demonstrated that mediolateral head motion during cycling can be used to estimate core stability status, as determined by the SCST.13 The poor core stability group, as defined by the SCST, exhibited greater laterally asymmetrical head motion than the good core stability group during cycling. The reaction force generated by pedaling causes lateral movement of the trunk. Due to the deficiency in core stability, postural stability is not maintained against the reaction force,14–16 which results in an imbalance of the mediolateral head motion. Measuring head motion in the frontal plane during cycling could serve as an alternative way to evaluate core stability, without the need for an additional examiner.
Seated hip flexion motion could offer an alternative way to evaluate core stability. Because seated hip flexion motion is a cycling motion, the head motion arising in response to seated hip flexion would be similar to that in response to cycling. The magnitude of lumbar motion arising from hip flexion might depend on the core stability. Core muscle activity occurs prior to distal extremity movement to maintain postural stability.6,17 The inability to control core muscle activity would lead to different lumbar and head motions between left and right leg hip flexion.
Since seated hip flexion is an easier task than cycling, it could serve as an alternative user-friendly core stability test. Thus, the aim of this study was to investigate whether seated hip flexion can be used as an alternative indicator of core stability. Our study participants were divided into good and poor core stability groups based on their SCST scores. We then compared the asymmetry in mediolateral head motion during seated hip flexion between the two groups. We hypothesized that the poor core stability group would show greater asymmetry in mediolateral head motion during seated hip flexion compared with the good core stability group, and that the magnitude of asymmetry would be similar between seated hip flexion and cycling tests.
METHODS
This study included 75 subjects (30 males and 45 females; mean age, 23.0±2.2 years; mean height, 166.4±8.9 cm; mean weight, 62.5±13.3 kg) (Table 1). We excluded pregnant individuals, as well as those with vestibular, neurological, cardiopulmonary, or psychological disorders, and musculoskeletal pathologies. All participants provided data on demographic characteristics and completed a visual analogue scale (VAS) pain score on daily activity and cycling. Participants who had a VAS score > 3 when cycling were also excluded from this study. Anthropometric data (height and weight) were measured using a measuring tape and electronic scale. Written informed consent was obtained from all participants. This study was approved by the Institutional Review Board of University.
To evaluate core stability, all participants underwent the SCST, which includes five progressively more difficult tasks. The tasks were performed as reported previously.10 The participants were placed in a crooked lying position, with the inflatable pad of a stabilizer pressure biofeedback unit (Chattanooga Group, Hixson, TN, USA) placed in the natural lordotic curve. The pad was inflated to 40 mmHg, and the participants were asked to draw in their abdomen for maintaining the pressure. A deviation > 10 mmHg was considered as loss of lumbar stabilization. If the participant completed an SCST task without deviation > 10 mmHg, they were then instructed to perform the next task. After four to five familiarization trials, the ability of the participants to complete the tasks without deviation > 10 mmHg was assessed using a 5-point scale. Based on the SCST score, the participants were divided into poor and good core stability groups (SCST cores of 0–1 and 2–5, respectively).
Head angle was measured with a left wireless earbud (QCY-T1C; Dongguan Hele Electronics, Dongguan, China) that included a high-resolution single inertial measurement unit (IMU; BNO080; Ceva Technologies, Rockville, MD, USA) equipped with a triaxial accelerometer and triaxial gyroscope (Figure 1A). Each IMU data sample had 16-bit acceleration for the x, y, and z axes. The IMU data were then transferred to a self-developed mobile app via a Bluetooth connection at 100 Hz. The mediolateral head angle was calculated in real time by the app.18
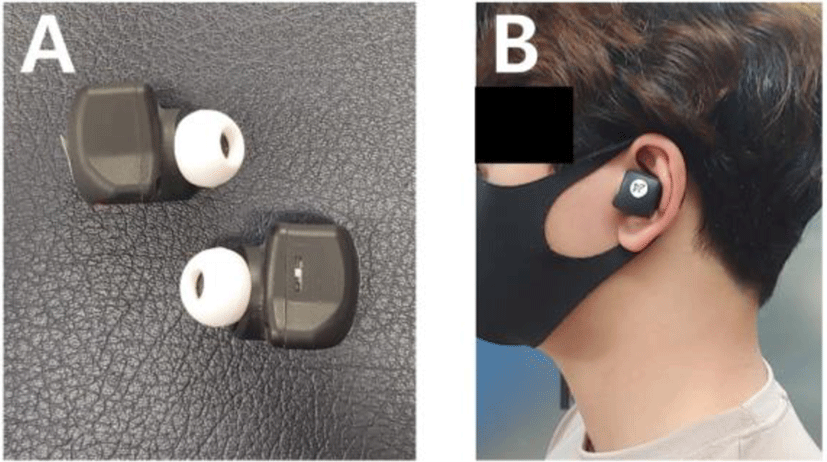
The mediolateral head angle was measured during the seated hip flexion test using the wireless earbud and IMU sensor. To minimize the effect of starting posture on the mediolateral head angle during hip flexion, we asked the participants to assume an identical starting posture. The participants were instructed to sit on a stool with the hips flexed at 90° (Figure 2A). The height of the stool was adjusted such that the participants could not contact the floor with their feet. The participants were asked to vertically align the external meatus, acromion, and greater trochanter, cross and place their arms on their chest, and look straight ahead (Figure 2A). The target bar was placed above the knee, which indicates 10° hip flexion relative to the starting position. Then, after positioning the wireless earbud, the participants were asked to raise the dominant or non-dominant leg with no knee extension at a comfortable speed, until the distal end point of the femur reached the target bar with 100° of hip flexion. Mediolateral head motion was measured until the dominant or non-dominant leg was returned back to the starting position after touching the target bar. Both the dominant and non-dominant leg were tested, and the order of testing on the legs was random.
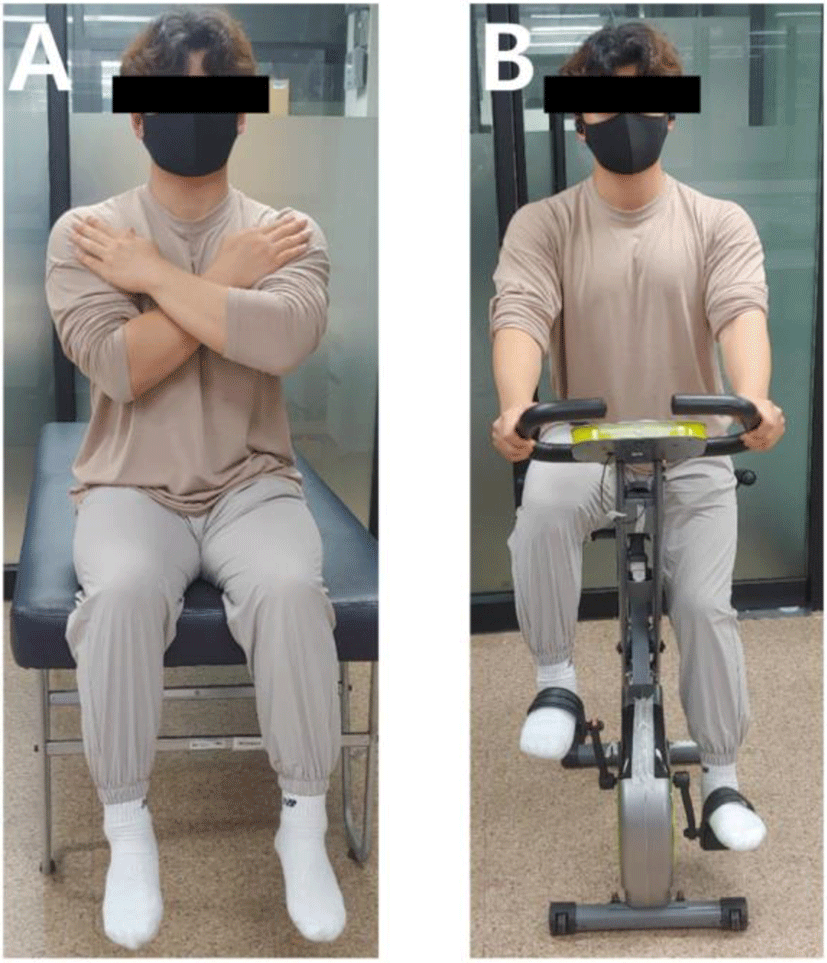
The participants performed cycling for about 1 min while wearing a left wireless earbud equipped with an IMU sensor (Figure 2B). Through the IMU sensor in the wireless earbud, the mediolateral head motion was measured during cycling. Before the test trial, the participants were allowed to perform a 5-min warm-up cycle to familiarize themselves with the cycling. After a 5-min rest period, the maximum cycle speed was measured, to set the target speed as 70% of the measured maximum speed. The participants were then asked to cycle at the target speed for 1 min. The mediolateral head angle was measured during cycling. Only the final 40 s of cycling was used in the data analysis (Figure 3).
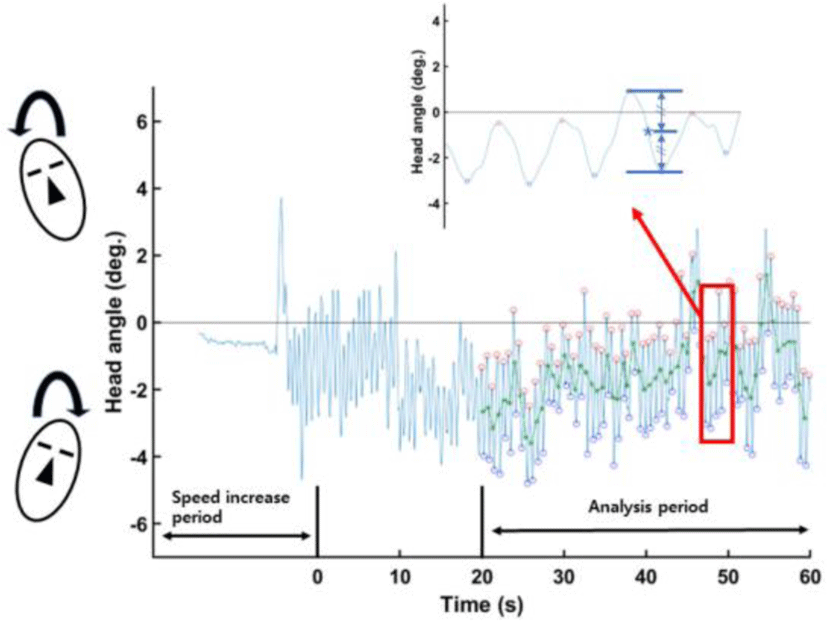
To evaluate symmetry in the head angle during cycling, the maximal right and left head angles were noted for each cycle. The positive and negative signs for the mediolateral head angle represent the left and right directions, respectively. The maximum (minimum) head angle corresponded to the left (right) maximal head angle.
The average of the maximal and minimal values of each cycling cycle corresponded to the mediolateral head angle symmetry. The average across all cycles was considered to denote the mediolateral head angle symmetry during the entire analysis period:
where maxi and mini represent the maximal and the minimal value of the ith cycle, respectively, and n is the total number of cycling cycles. The unit for SIcycle is “degrees”. An SI of 0° indicates a perfectly symmetric mediolateral head angle, as the values for each direction have opposite signs (Figure 3).
The maximum right angle was picked during the left seated hip flexion test and the maximum left angle was picked during the right seated hip flexion test. Similar to the cycling setup, the positive and negative signs represent the left and right directions, respectively. The mediolateral head angle symmetry was calculated by averaging the maximal value of the right hip flexion test and minimum value of the left hip flexion test:
Here maxrt and minlt are the maximal mediolateral head angle during the right seated hip flexion test and minimal mediolateral head angle during the left seated hip flexion, respectively. The unit for SIhfl is degrees. Similar to SIcycle, the closer the SIhfl value is to 0°, the more symmetric the head angle is in the frontal plane (Figure 4).
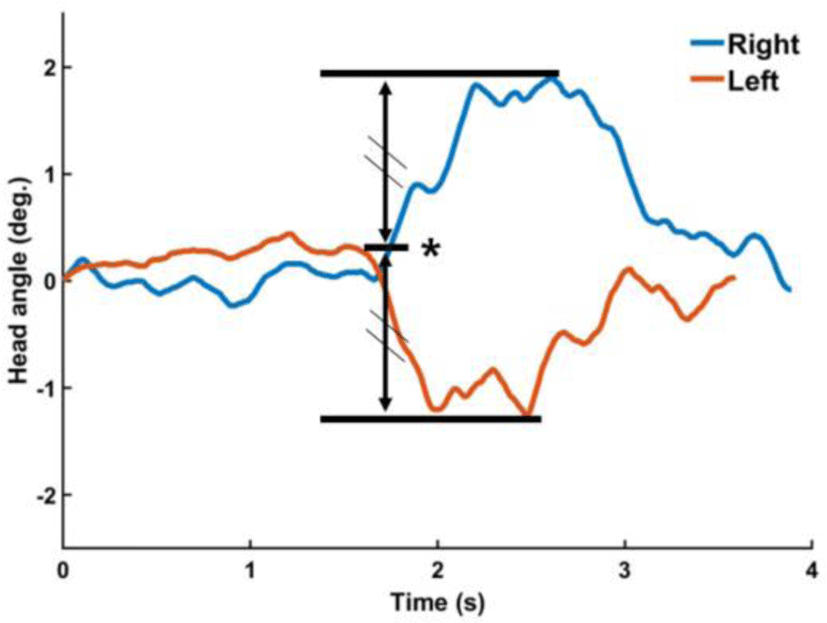
To test the effects of core stability on symmetry in mediolateral head motion during indoor cycling and the seated hip flexion test, an independent t-test was used to compare SIcycle and SIhfl between the poor and the good core stability groups in MATLAB (Mathworks, R2022a, Natick, MA). The significant difference in SIcycle and SIhfl between the poor- and the good-core-stability group was considered as the significant effect of core stability on SIcycle and SIhfl. The level of statistical significance was set at 0.05.
RESULTS
Of the 75 participants, 27 obtained SCST scores of 0–1 and were assigned to the poor core stability group; the other 48 participants (SCST scores > 1) were assigned to the good core stability group. No significant group differences were observed in age, height, weight, BMI, or maximal cycle speed. The VAS for pain in the poor core stability group was significantly higher than in the good core stability group (Table 1). However, all participants completed the SCST, cycling test, and seated hip flexion test with no pain.
During the cycling test, the symmetry index (SIcycle) in the poor core stability group was significantly higher than in the good core stability group (Figure 5A). Because less SIcycle coincides with better symmetry, the good core stability group showed more symmetrical mediolateral head motion than the poor core stability group. In contrast, there was no significant difference in the symmetry index during seated hip flexion (SIhfl) between the poor and good core stability groups (Figure 5B).
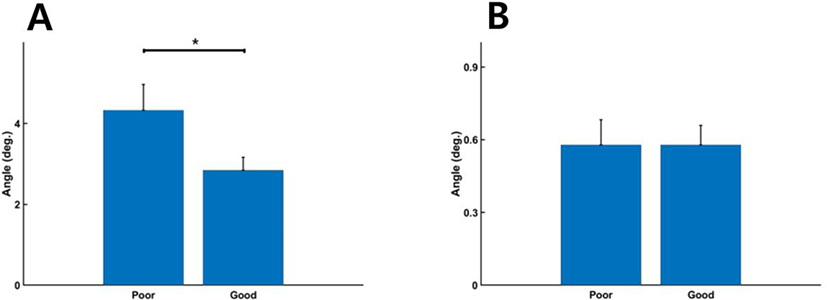
DISCUSSION
The purpose of this study was to investigate whether mediolateral head motion during the seated hip flexion test is affected by core stability status. Mediolateral head motion in individuals with a low SCST score was more asymmetrical during the cycling test compared to individuals with a high SCST score; and that the symmetry in the mediolateral head motion during seated hip flexion was not significantly different between the poor and good core stability groups.
The SCST evaluates the asymmetry in trunk motion during rhythmic movements, as well as the ability to control the lumbopelvic segment. To achieve a high SCST score, the deep muscles of the multifidus and transverse abdominis, the so-called ‘core muscles’, should be properly controlled to maintain lumbar curvature against lower extremity movements.10,19 Individuals with a low SCST score are characterized by dysfunctional control of the core muscles. Poor control of the core muscles could cause asymmetric activity thereof, which can give rise to bilaterally asymmetric movements. Our poor core stability group had greater asymmetry in mediolateral head motion during the cycling test than the good core stability group (Figure 5A). This result supports the notion that poor core stability induces asymmetry in postural stability for distal mobility.
Mediolateral head motion partially reflects mediolateral trunk motion during rhythmic movements, such as walking or cycling. A previous study demonstrated that the mediolateral head and trunk motion influence each other; specifically, restricting either motion affects the other during gait motion.20 Furthermore, core stability is associated with torso stability during cycling.4,9 Reduced core stability lowers torso stability, resulting in greater mediolateral trunk motion. Similar to trunk motion, mediolateral head motion in our poor core stability group was larger than in the good core stability group during the cycling test (Figure 5A). These findings suggest that head motion is partially related to trunk motion during rhythmic movements.
There was no significant difference in the symmetry of mediolateral head motion between our poor and the good core stability groups when performing the seated hip flexion test. The seated hip flexion test has several similarities to cycling; both require hip joint movements in a sitting position and both limit upper extremity movements. However, the difference in core stability did not significantly influence symmetrical kinematics during the seated hip flexion test. The participants were instructed to raise their leg at a comfortable speed during the hip flexion test. The average time to reach the maximal right or left head angle was longer than the time needed during the cycling test. Core stability can be understood as the “preprogrammed integration” of muscles located at a lumbopelvic segment.2,4 The slower speed could not invoke the preprogrammed integration for proximal stability. Therefore, the participants voluntarily controlled a lumbopelvic segment, which resulted in different results between the seated hip flexion and cycling tests.
Different movement strategies could explain the discrepancy between seated hip flexion and cycling test results. During the seated hip flexion test, the participants flexed the hip by 10° without any external resistance. Trunk motion in the hip flexion test depends on hip flexibility and strength, as well as core stability.21 An insufficient range of hip motion or weak hip flexor strength could lead to compensative movement in the lumbopelvic segment. In this case, trunk motion may occur to accomplish the required hip flexion, but not to maintain postural stability against lower extremity movement. That is, the trunk motion in the seated hip flexion test is not related to core stability. In contrast, the trunk motion during cycling is strongly associated with core stability. The reaction force generated by pedaling is transferred to the upper body, which causes the trunk to move laterally. To maintain postural stability, the preprogrammed core muscle activity reduces trunk lateral motion.2,6,9,22,23 Thus, the difference in movement strategy can explain the different results.
A wireless earbud equipped with an IMU sensor can estimate core stability status during cycling. Appropriate evaluation of core stability is essential to reduce the risk of injury. Core stability is related to low back pain and lower extremity injury.6,7,24–26 If people are able to recognize their core stability status, they can work towards promoting or maintaining it. In this study, individuals with poor core stability produced more asymmetrical mediolateral head motion than those with good core stability. This suggests that simple measurement of mediolateral head motion can be used to evaluate core stability. Compared to the SCST requiring a well-trained examiner, this approach is easier and more applicable, where it is possible to measure core stability status with no examiner.
Further research is needed to determine the optimal angle of asymmetry for assessing core stability status. In this study, the poor core stability group had greater asymmetry in mediolateral head motion than the good core stability group. Additionally, a previous study reported that head motion is associated with trunk motion during rhythmic movements, but the exact relationship between head and trunk motion has rarely been studied. To clarify whether head mediolateral motion represents trunk motion, further work will be necessary to evaluate their association during cycling.
CONCLUSION
Mediolateral head motion during indoor cycling showed greater asymmetry in the poor-core-stability group than in the good-core-stability group. However, symmetry in mediolateral head motion during the seated hip flexion test did not significantly differ between the poor-core-stability group and the good-core-stability group. These results suggest that indoor cycling can be an alternative method to evaluate core stability status, but not the seated hip flexion test.